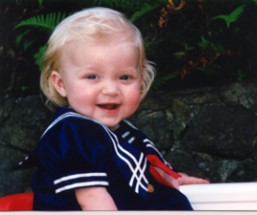
I envision that a few years from now when I have a patient waiting for an organ, I could pick the organ from the shelf and save this patient’s life.
Carlos Esquivel, M.D., Ph.D.; Professor of Surgery; Director Pediatric Liver Transplant Program; Chief, Division of Abdominal Transplantation, Stanford University
Nearly thirty years ago, Dr. Esquivel performed a liver transplant on our one-year old daughter. Otherwise healthy, Clare suffered from biliary atresia, a rare pediatric disease in which the bile ducts are malformed and do not drain properly. While Clare’s first liver transplant was initially successful, it subsequently failed as the donor liver came from a younger baby whose hepatic vessels were too small for a one-year old. A national emergency call went out for a second cadaver liver, this time procured from a 10-year who had died in a terrible accident playing in his back yard. That transplant was successful; our next hurdle was to manage rejection with a promising new immunosuppression drug. We thought we were out of the woods. But then we drew the unlikeliest and unluckiest of outcomes: unbeknownst to the doctors at the time, some young children are genetically pre-disposed to contracting a wild lymphoma-like disease from anti-rejection drugs. Despite her well-functioning new liver, Clare unexplainably died some months later.
Recently described by one Mass General surgeon as a sophisticated but straightforward plumbing operation, organ transplantation is now practiced in more than 250 medical centers in the U.S. It is big business, but it is heavily supply constrained. Roughly 40,000 organ transplants were performed in the US in 2020, the vast majority from cadaver organs. Many patients die each year before a suitable organ becomes available—there are more than 100,000 patients on the waitlist and likely another 100,000 candidates who do not have access to informed health care that would allow them to join the waitlist. While managing post-transplant rejection (the biggest cause of transplant failure) has improved with new drug regimens, much of the process we experienced — anxious waiting as the patient deteriorates, uncertainties of waitlist matching, and undergoing a transplant with a cadaver organ — has not changed.
The methods of producing and procuring replacement organs will undergo dramatic change in the next decade. Through our 2032 class discussions of CRISPR-Cas9 genome editing, the Broad Institute, Twist Bioscience and Ginkgo — we have gotten a glimpse of technology and tools that may alleviate the organ shortage and eventually make Dr. Esquivel’s vision of off-the-shelf organs a reality, maybe not by 2032, but perhaps shortly after.
in January of this year, a pig was genetically engineered for the first xenotransplantation of an animal heart into a human. Thanks to CRISPR gene editing at Revivicor, the “donor” pig underwent 10 genetic modifications to reduce the likelihood of rejection. Three pig genes that might cause rejection were eliminated and six were added to enhance organ acceptance. Another gene modification suppressed the growth of the pig heart so as to maintain an appropriate size for humans.
Sadly, the recipient patient, who had suffered from severe heart failure, died two months after the xenotransplant; a detailed analysis of the cause of death is yet to be published. Despite this setback, it is likely that we will see continued progress and successful xenotransplants of kidneys, hearts and probably livers well before 2032. (At NYU, kidneys from modified pigs which only required one gene edit have been transplanted into legally deceased patients with no brain function; the deceased recipients did not reject the organs while they were sustained on ventilators).
Fraught with complex technical, environmental, and ethical issues, engineering animal organs and tissues for transplant is a stop-gap measure at best. Further out—hopefully before 2042– Dr. Esquivel’s vision will be realized with tissue-engineered organs. Grown on a bio compatible scaffold, these 3-D printed organs may be engineered with induced pluripotent stem (iPS) cells that are friendly to the recipient, eliminating or reducing the rejection problem. Needless to say, designing effective scaffolding material and the creation of complex vascular structures are daunting tasks.
The development will be leveraged through the use of bio 3-D printing and common platforms to grow different types of tissue—skin (which has already been produced and tested in humans), organs, cartilage, etc. Since tissue engineering offers broader applications and relies on more widely-used technologies such as 3-D printing, it will enjoy more rapid cost reduction than engineering animal organs.
In 2032, and certainly in 2042, we will look back and think how primitive organ transplants were at the turn of the millennium. Of course, off-the-shelf organs will introduce new ethical and social issues. But despite such challenges, having lived through a pre-millennium transplant, I am enthusiastic about the technologies that will realize Dr. Esquivel’s vision.
Mary Sauer is a senior fellow in the Harvard Advanced Leadership Initiative. She co-founded, oversaw an IPO, and then sold Sonic Solutions, a digital media software company that won an Emmy for digital audio signal processing technology used to remove noise from analog recordings for release on digital formats. Her personal passions include the art, social and economic life of Renaissance Italy and summer sailing with her family.
0 Comments